Living in cities is obviously very comfortable, as all types of goods and services are available to us. One of the perhaps most underestimated luxuries in this regard is green infrastructure in cities and other urban areas. This green infrastructure includes everything from parks, private gardens, and trees lining roads to green roofs and walls – essentially, anything that helps to absorb, delay, and treat stormwater, thereby mitigating flooding and pollution downstream. At the same time, green infrastructure offers significant aesthetic value, and it also absorbs carbon dioxide, thus helping to reduce our carbon footprint. A single large, healthy tree can remove more than 150 kg of carbon dioxide from the atmosphere each year. Finally, greenery increases property values, especially in the immediate vicinity of parks and other green spaces. Urbanized areas act as heat islands that experience higher temperatures than their surroundings. Man-made structures such as buildings and roads absorb and re-emit the sun’s heat more than natural landscapes such as forests and bodies of water. For this reason, trees and urban forests are essential in built-up areas: They provide numerous ecosystem services and make our lives easier and more pleasant. In addition, they can help to lower energy consumption and reduce energy costs for heating and cooling: Trees placed around homes to shade windows result in high energy savings. They also provide evaporative cooling through their leaves, which increases air humidity. Shaded areas are cooler, and evapo-transpiration can lower peak summer temperatures by two to nine degrees. And in cities that have considerable problems with flooding, trees can mitigate this risk since they intercept around a third of the rainfall that hits them. Urban trees also reduce particulate matter less than 10 micrometres in diameter (PM 10) by 0.1 kg per tree per year, and they diminish ozone and sulphur dioxide as well. All of these ecosystem services could be enhanced by increasing tree density.
From the point of view of managing tree species, it is very important for each city to have its own green infrastructure strategy and plan to implement. It is obvious how essential trees are and will be in the future for coping with and adapting to difficult climate changes. Changing climate factors – and especially the occurrence of droughts – will dramatically affect tree habitats in cities. For most tree species, the urban environment already represents an extreme habitat with limited growing space, shallow soil, nutrient deficiency, higher soil and air temperatures, and the presence of salt and pollutants. Trees are also often exposed to various forms of mechanical damage in city environments. Therefore, urban trees should be selected for their durability and resilience, character, growth habit, and aesthetic value. Urban forests should feature a diversity of species and avoid monocultures, which are less resistant to pests and other environmental factors that can damage or kill trees.
Management of NNT in the urban area
Sustainable management and use of non-native tree species (NNT) in urban areas implies several steps, including identification of suitable tree species and their long-term management. The main aim of such designed concept is to prevent disruption of ecosystem integrity, while improving the resilience of urban areas to environmental constraints and enhancing an overall ecosystem functionality, in parallel.
Identification of suitable NNT for growing in urban areas imposes itself as a priority, since the correct choice of species may prevent potential problems in the future and help achieving planting objectives. Although NNT have been widely planted in Europe since middle age, there is a controversy about the use of NNT in the future. In general, the main concerns for the use of NNT in urban areas are related to their possible invasion risks and putative inferiority to locally adapted native species. Therefore, NNT has to meet a broad spectrum of criteria to be considered for planting in urban settlements. Some of them are listed below:
- aesthetic quality
- rooting ability and depth
- growth rate
- trees allergenic properties
- drought tolerance
- pest and diseases tolerance
- salt tolerance (e.g., in case of tree species planted along the roads)
- do not require fertilizers
- to require little long-term maintenance (e.g., frequent pruning)
- to be non-invasive
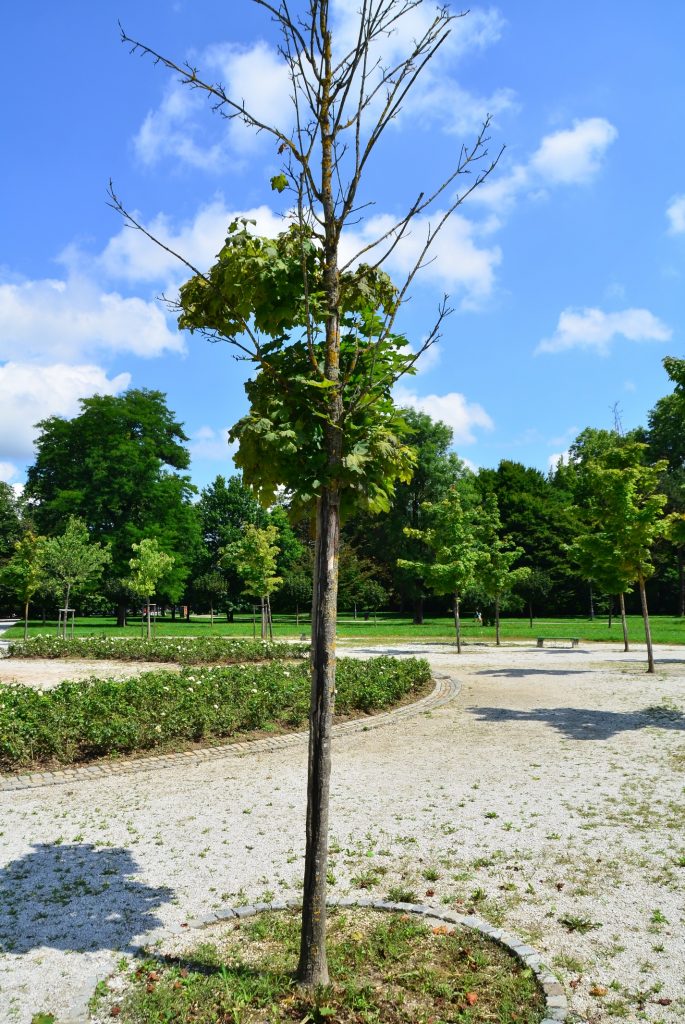
Urban trees generate significant ecosystem services, such as carbon sequestration, removing air pollutants, regulating the microclimate, thus contributing to overall improvement of environmental quality and quality of life, accordingly. However, selecting the right species is a challenge, as the most guidance is focused on trees aesthetic values and ecosystem service delivery, very often neglecting the importance of trees ability to cope with different biotic and abiotic stress factors. Additional challenge for urban foresters is to target the most appropriate tree species for selected urban sites under projections of future climatic conditions, imposing the need for understanding the species climate-adaptation properties and environmental stress tolerance.
So far, a solution has been sought mainly in the use of suitable indigenous species and local provenances, while the use of NNT was significantly burdened by the negative public perception, although numerous studies demonstrated higher drought tolerance of NNT growing in urban areas compared to autochthonous species. Nevertheless, in order to mitigate negative effects of climate change and maximize ecosystem services, the use of urban trees should not rely only on the planting of autochthonous tree species, but should also consider selection of other provenances and NNT.
Drought resistance of non-native trees
Unlike trees growing in natural communities, urban trees are subjected to additional human influences. They therefore struggle with complex stresses that are less present or even non-existent in forest ecosystems. These stresses commonly act simultaneously or sequentially, chaining a large pool of negative factors. For example, soil compaction caused by human activity reduces the capacity of urban soils to absorb water, inducing a water deficit in trees that in turn can deteriorate their vitality, making them more vulnerable to pest and disease outbreaks. Likewise, it is well documented that certain factors can generate both growth-stimulating and stress-inducing conditions at once. For example, elevated CO2 concentrations increase the photosynthesis rate in plants, leading to faster growth and production of above-ground biomass. However, increased CO2 concentration also decreases the transpiration rate, causing reducing convective heat loss and exposing plants to a higher risk of overheating. Therefore, depending on the intensity and duration of these stresses, the health and growth rate of trees can be significantly reduced.
Moreover, as a consequence of the harsh environmental conditions in cities, the lifespan of urban trees is frequently shorter than that of trees growing in natural habitats. Although the causes of urban tree decline are complex and involve various direct and indirect factors, drought stress has been determined as the main driver responsible for damaging trees in urban areas. Drought stress occurs in plants exposed to low soil water availability and atmospheric conditions causing high evaporative demands. In terms of time scale, drought can be either short-term or long-term. Short-term drought usually lasts between a few weeks and a few months and primarily affects young and newly transplanted trees that have not yet developed extensive root systems.
Tree species display a range of strategies for withstanding drought stress, from physiological and biochemical responses to modulation of their anatomy and morphology. Physiological and biochemical adjustments occur inside plant cells and organs and commonly include stomatal control of water loss, reduction of leaf water potential, and maintenance of water uptake through osmotic adjustment within the cells. However, these mechanisms seem to depend largely on drought progress and its severity: Under conditions of mild to moderate drought, stomatal closure is the major physiological response mechanism, with the consequence of decreased CO2 availability in the mesophyll and reduction of the photosynthetic rate. By contrast, however, stomatal control of photosynthesis seems to become progressively less effective as drought stress intensifies, and non-stomatal (i.e., biochemical and metabolic) limitations to CO2 assimilation begin to prevail. Furthermore, morphological and anatomical alterations such as reduction of leaf area, increase of leaf sclerophylly, and development of prolific and deep root systems have been recognized as key components of tree acclimatization to long-term drought stress. As the drought resistance of trees is commonly based on two main strategies, namely drought avoidance and drought tolerance, different tree species may have widely contrasting responses to drought-induced stress. Bearing these considerations in mind, the selection of suitable tree species for cultivation in urban areas should rely not only on their aesthetic qualities but also on an understanding of the complex interaction between the physiology of plants and their surrounding environment.
Taking the socio-ecological aims of urban forests into account as well, the selection of multifunctional tree species should be oriented toward mitigating the negative effects of climate change and maximizing provided ecosystem services. In this sense, both inter-and intra-specific diversity should be promoted to enhance the resilience of urban forests to environmental pressures and ensure the continued provision of functions relating to human well-being as well as landscape preservation and beautification. Introducing non-native tree species (NNT) alongside drought-resistant autochthonous species has been identified as a possible solution for increasing species diversity in cities. Although there is widespread concern that biodiversity loss may occur as a consequence of harmful effects of NNT on native species, a recent study conducted in the UK showed that 75% of respondents would be happy to see more non-native planting in UK public spaces if the selected species were better adapted to the changing climate than existing vegetation. It is therefore likely that the relevance of NNT will increase under future climate change conditions since public awareness regarding their role in securing the resilience of urban tree populations is rising.
Accordingly, a number of studies have discovered evidence of higher drought tolerance of NNT growing in urban areas as compared to autochthonous species. For example, a dendroecological study conducted on several tree species cultivated along the streets of Dresden (Germany) showed that Quercus rubra and Platanus x hispanica are better adapted to warm and dry conditions than Acer platanoides and Acer pseudoplatanus. Similarly, Vaštag et al reported that Acer platanoides trees growing in the urban area of Novi Sad, Serbia exhibited a more severe reduction in physiological performance than Liquidambar styraciflua when subjected to drought stress.
Moreover, as the stresses encountered by urban trees depend on growing conditions in urban areas (e.g., park trees and trees growing in urban woodlands are less affected by stress factors than trees occurring on squares, in tree pits, or along roads), a variety of tree species including NNT and less commonly used autochthonous species should be considered for planting in urban environments. In this context, comprehensive guidance on the drought tolerance and growth potential of different tree species in various urban conditions should be developed for specific regions, including a list of traits that could be exploited as bio-indicators of those species’ resistance to stresses.
Further resources:
PODCAST – Stress in the City
For trees to be able to provide ecosystem services in cities, trees have to be respected as living creatures and not treated as decorations for parking lots. Hot temperatures, drought, air pollution, noise, light pollution, and isolation take a toll on the health and wellbeing of all species under extreme circumstances. Available at: https://youtu.be/v2jRNwEfDtk
Report on Urban NNT assessment in the Alpine Space
Urban trees are more vulnerable to climate change due to more extreme climatic environments, necessitating adaptive measures in tree selection, which increasingly makes non-native trees potentially suitable candidates for urban applications in the future. With this report, we aim to fill some knowledge gaps by assessing non-native urban tree species drought tolerance to provide technical recommendations for urban and peri-urban green infrastructure. The results of this activity will support the data modeling activity, as well as recommendations for growing non-native tree species (NNT) in urban areas.Available at: deliverable-d-t2-3-1-report-on-urban-nnt-assessment.pdf
Scientific References
1. Swoczyna, T., Kalaji, H.M., Pietkiewicz, S., Borowski, J. Ability of various tree species to acclimation in urban environmentsprobed with the JIP-test. Urban Forestry & Urban Greening 14, 544-553 (2015).
2. McDermot, C.R., Minocha, R., D’Amico III, V., Long, S., Trammell, T.L.E. Red maple (Acer rubrum L.) trees demonstrate acclimation to urban conditions in deciduous forests embedded in cities. PLoS ONE 15, 7. e0236313 (2020).
3. Michael, T., Dananjali, G., Naoki, H., Anke, A., Saman, S. Effects of elevated carbon dioxide on photosynthesis and carbon partitioning: a perspective on root sugar sensing and hormonal crosstalk. Frontiers in Physiology 8, 578 (2017).
4. Katul, G., Manzoni, S., Palmroth, S., Oren, R. A stomatal optimization theory to describe the effects of atmospheric CO2 on leaf photosynthesis and transpiration. Annals of Botany 105, 431-442 (2010).
5. Wright, I.J., Ning Dong, N., Maire, V., Prentice, C.I., Westoby, M., Díaz, S., Gallagher, R.V., Jacobs, B.F., Kooyman, R., Law, E.A., Leishman, M.R., Niinemets, Ü., Reich, P.B., Sack, L., Villar, R., Wang, H., Wilf, P. Global climatic drivers of leaf size. Science 357, 917. DOI: 10.1126/science.aal4760 (2017).
6. Whitlow, T.H., Bassuk, N.L., Reichert, D.L. A 3-year-study of water relations of urban street trees. Journal of Applied Ecology 29, 2: 436-450 (1992).
7. Kleerekoper, L., Van Esch, M., Salcedo, T.B. How to make a city climate-proof, addressing the urban heat island effect. Resources, Conservation and Recycling 64, 30-38 (2012).
8. Sjöman, H., Hirons, A.D., Bassuk, N.L. Urban forest resilience through tree selection – variation in drought tolerance in Acer. Urban Forestry & Urban Greening 14, 858-865 (2015).
9. Gilbertson, P., Bradshaw, A.D. The survival of newly planted trees in inner cities. Arboricultural Journal: The International Journal of Urban Forestry 14, 287-309 (1990).
10. Nitschke, C.R., Nichols, S., Allen, K., Dobbs, C., Livesley, S.J., Baker, P., Lynch, Y. The influence of climate and drought on urban tree growth in southeast Australia and the implications for future growth under climate change. Landscape and Urban Planning 167, 275-287 (2017).
11. Cameron, R.W.F. Plants in the environment: amenity horticulture. In: Thomas, B., Murphy, D., Murray, B. (Eds.), The Encyclopedia of Applied Plant Sciences. Elsevier Science Ltd, London, pp. 735-742 (2003).
12. Kesić, L., Vuksanović, V., Karaklić, V., Vaštag, E. Variation of leaf water potential and leaf gas exchange parameters of seven silver linden (Tilia tomentosa Moench) genotypes in urban environment. Topola/Poplar 205, 15-24 (2020).
13. Thomas, F.M., Gausling, T. Morphological and physiological responses of oak seedlings (Quercus petraea and Q. robur) to moderate drought. Annals of Forest Science 57, 325-333 (2000).
14. Deligöz, A., Bayar, E. Drought stress responses of seedlings of two oak species (Quercus cerris and Quercus robur). Turkish Journal of Agriculture and Forestry 42, 114-123 (2018).
15. Sanders G.J., Arndt S.K. Osmotic adjustment under drought conditions. In: Aroca R. (eds) Plant responses to drought stress. Springer, Berlin, Heidelberg (2012).
16. Escalona, J.M., Flexas, J., Medrano, H. Stomatal and non-stomatal limitations of photosynthesis under water stress in field-grown grapevines. Functional Plant Biology, 27, 1: 87-87 (2000).
17. Gallé and Feller, 2007[AA1]
18. Farooq M., Wahid A., Kobayashi N., Fujita D., Basra S.M.A. Plant drought stress: effects, mechanisms and management. In: Lichtfouse E., Navarrete M., Debaeke P., Véronique S., Alberola C. (eds). Sustainable Agriculture. Springer, Dordrecht (2009).
19. Stojnić, S., Kovačević, B., Kebert, M., Vaštag, E., Bojović, M., Stanković-Neđić, M., Orlović, S. The use of physiological, biochemical and morpho-anatomical traits in tree breeding for improved water-use efficiency of Quercus robur L. Forest Systems 28, 3. e017 (2019).
20. Sjöman, H., Hirons, A. D., Bassuk, N. L. Improving confidence in tree species selection for challenging urban sites: a role for leaf turgor loss. Urban Ecosystems 21, 1171-1188 (2018).
21. Ferrini, F., Bussotti, F., Tattini, M., Fini, A. Trees in the urban environment: response mechanisms and benefits for the ecosystem should guide plant selection for future plantings. Agrochimica 58, 3: 234-246 (2014).
22. Kendal, D., Dobbs, C., Lohr, V.I. Global patterns of diversity in the urban forest: Is there evidence to support the 10/20/30 rule? Urban Forestry & Urban Greening 13, 411-417 (2014).
23. Schlaepfer, M.A., Guinaudeau, B.P., Martin, P., Wyler, N. Quantifying the contributions of native and non-native trees to a city’s biodiversity and ecosystem services. Urban Forestry & Urban Greening 56. 126861 (2020).
24. Chalker-Scott, L. Nonnative, noninvasive woody species can enhance urban landscape biodiversity. Arboriculture & Urban Forestry 41(4): 173-186 (2015).
25. Hoyle, H., Hitchmough, J., Jorgensen, A. Attractive, climate-adapted and sustainable? Public perception of non-native planting in the designed urban landscape. Landscape and Urban Planning 164, 49-63 (2017).
26. Gillner, S., Bräuning, A., Roloff, A. Dendrochronological analysis of urban trees: climatic response and impact of drought on frequently used tree species. Trees-Structure and Function 28, 1079-1093 (2014).
27. Vastag, E., Kesić, L., Orlović, S., Karaklić, V., Zorić, M., Vuksanović, V., Stojnić, S. Physiological performance of sweetgum (Liquidambar stryraciflua L.) and norway maple (Acer platanoides L.) under drought condition in urban environment. Topola/Poplar 204, 17-27 (2019).
28. Swoczyna, T., Borowski, J., Pietkiewicz, S., Kalaji, H.M. Growth and physiological performance of young urban trees of eight taxa in Warsaw. Plants in Urban Areas and Landscape. Slovak University of Agriculture in Nitra. p. 15-19. ISBN: 978-80-552-1262-3 (2014).
29. Czaja, M., Kołton, A., Muras, P. The complex issue of urban trees – stress factor accumulation and ecological service possibilities. Forests 11, 9: 932 (2020).